Boosting oxygenation for transplanted cell-based therapies
To advance cell-based therapies, researchers have identified a novel device that makes on-site oxygen for biological cells transplanted inside the body.
Cell-based therapies, which use biological cells to treat diseases or injuries in the body, offer enormous potential from facilitating drug delivery to enabling more effective treatment of obesity, diabetes, and cancer. Generating on-site oxygen is a critical component of activating such therapies, because there is a high metabolic demand on these densely packed cells, and maintaining cell potency becomes a challenge, due to factors like immune response and dissolved oxygen. As part of an ongoing, multidisciplinary collaboration, a group of researchers from Carnegie Mellon University, Northwestern University, Georgia Institute of Technology and Rice University have identified a novel device that makes on-site oxygen for biological cells transplanted inside the body.
Every cell is closely situated to tiny blood capillaries due to its need of sufficient oxygen supply to function. Current approaches to generating on-site oxygen for cell-based therapies have drawbacks including size and supply capacity. Some options introduce gaseous oxygen from outside the body to tackle the problem but are bulky; others are limited by supply capacity, like a can of water that cannot be refilled once it’s been emptied. This new device boasts a compact footprint and relies on the abundance of water in the human body, making it an excellent choice for implantation, along with ensuring a sustainable and lasting source of oxygen.
Our device uses electricity and water to make oxygen for the cells—it’s as simple as a Chemistry 101 electrolysis experiment we all did as children, but we’re conducting it in a smarter manner.
Tzahi Cohen-Karni, Professor, Biomedical Engineering and Materials Science and Engineering
“Our device uses electricity and water to make oxygen for the cells—it’s as simple as a Chemistry 101 electrolysis experiment we all did as children, but we’re conducting it in a smarter manner,” said Tzahi Cohen-Karni, professor of biomedical engineering and materials science and engineering at Carnegie Mellon University. “We are using unique materials, including iridium oxide to produce oxygen, while avoiding the production of harmful byproducts, such as chlorine or excess hydrogen peroxide. By controlling how much electricity our device uses, we can change how much oxygen it produces.”
The biggest advantage and differentiator of the group’s research, recently published in Nature Communications, is that it bridges the gap between energy science, biomedical engineering, and electronics. Water splitting research, which is geared toward energy conversion and storage, guided exploration of which materials could safely be used for oxygen evolution reaction in the human body, which led to the group incorporating iridium oxide.
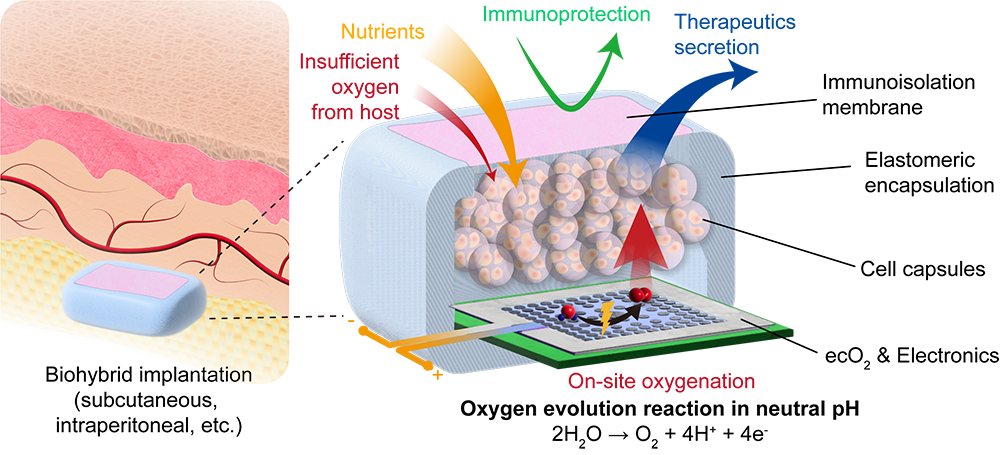
Source: Tzahi Cohen-Karni lab (CMU Engineering)
A schematic illustration of electrocatalytic on-site oxygenation.
“Unlike other approaches out there, we are using electrocatalysis, to reduce the energetic cost to generate the oxygen, and we are also tailoring the conditions of our device to provide oxygen without any byproducts, such as bleach,” added Inkyu Lee, materials science and engineering Ph.D. student. “We are also operating our device under conditions that will allow oxygen generation without bubbles that can disrupt the device.”
The cells in this study were biologically engineered to produce human leptin, a key component in the body’s mechanism for weight control and regulation of circadian rhythms, which is also being investigated by the group through the DARPA Normalizing Timing of Rhythms Across Internal Networks of Circadian Clocks (NTRAIN) project. The NTRAIN effort is managed by Jonathan Rivnay from Northwestern University.
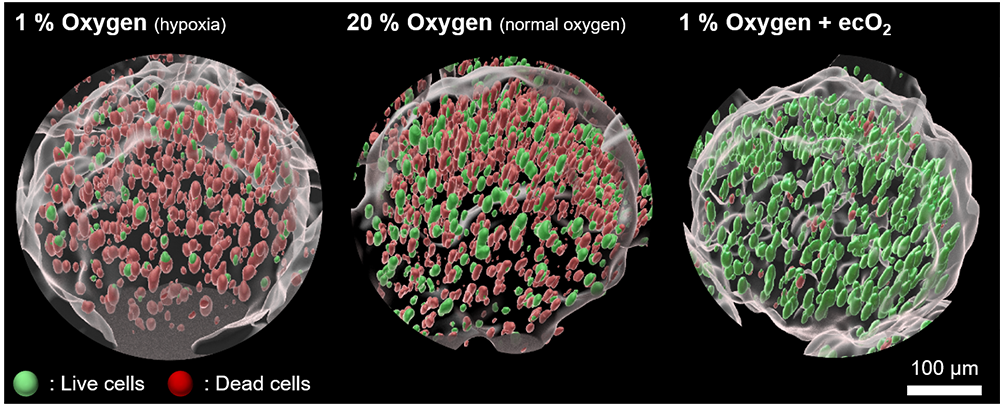
Source: Tzahi Cohen-Karni lab (CMU Engineering)
A representative set of 3D-rendered immunofluorescence images after 21 days in vitro oxygenation.
Additionally, some aspects of this published work will be used in the ARPA-H funded Targeted Hybrid Oncotherapeutic Regulation (THOR) project, which is combining the group’s expertise in an effort to develop sense-and-respond technology to treat ovarian, pancreatic and other difficult-to-treat cancers. The THOR projects is managed by Omid Veiseh from Rice University.
“We are the pioneers of this electrocatalytic oxygenation technology, and we have concrete plans to translate our devices to clinical practices in the real world,” emphasized Cohen-Karni. “We are not focusing on only one disease but instead, pushing to translate our work to various disease models.”
As this work moves forward, the team is concentrating on perfecting highly stable materials that can operate for months in the body and plan to deploy their cutting-edge approaches to treat chronic diseases.
The effort was co-led by Tzahi Cohen-Karni (MSE/BME, CMU) and Jonathan Rivanay (NU) and researchers Inkyu Lee (MSE, CMU) and Abhijith Surendran (NU). Other Carnegie Mellon participants involved in the research were Douglas Weber, professor of mechanical engineering; Adam Feinberg, professor of biomedical engineering; Sharon John, research associate; Seonghan Jo and Yingqiao Wang, materials science and engineering Ph.D. students; Tim Schwartzkopff, chemical engineering Ph.D. student; Omar El-Refy, physics Ph.D. student; and Ian Gimino, chemical engineering undergraduate student.