Magnetic symmetry is not just like looking in a mirror
Vincent Sokalski and Michael Kitcher have uncovered the quantitative explanation for magnetic symmetry breaking during domain wall motion, a contribution to the fundamental physics of technology needed to build faster computers.
When you think about how rapidly computers have filled our homes, our cars, and even ourselves through watches and earpieces, it might be hard to believe that there is a massive gap between a computer’s processing power and the speed, capacity, and reliability of our brains. But, by 2040, it is predicted that this gap will pose a critical problem for energy consumption, because by one estimate, all the energy produced in the world will be needed to meet the computational demands of our society.
The foundation of computer processing is memory, which is currently collected from different components. Long-term storage, like the 500 gigabytes on your laptop, often uses magnets, which are stable and cost effective. But short, random-access memory, the sort that is required when you load an application and must access it right away, doesn’t use magnetic memory. There is a large opportunity to magnetize this technology and reduce energy consumption, but in order to do so, researchers must better understand the behavior of one future magnetic memory concept: domain walls.
Vincent Sokalski has been studying the properties of domain walls, the magnetic defects that separate uniform magnetized regions. Sokalski and Michael Kitcher uncovered the quantitative explanation for magnetic symmetry breaking during domain wall motion based on experiments performed at the University of California San Diego using thin films of platinum, cobalt, and nickel.
Our concept of symmetry for magnetic materials is very different than our real-life concept of symmetry.
Vincent Sokalski, Associate Professor, Materials Science and Engineering
“Our concept of symmetry for magnetic materials is very different than our real-life concept of symmetry,” explained Sokalski, an associate professor of materials science and engineering. “Imagine you drop a blob of ink onto a piece of paper, you can expect that that blob will uniformly spread unless the paper is tilted to one direction. In that situation, you can expect that the blob will move in the direction of the tilt. With magnets, however, we found that this isn’t always the case.”
Rather than tilting a piece of paper, understanding domain wall symmetry requires applying magnetic fields to the material. Researchers at UCSD applied magnetic fields in various directions to Pt/Co/Ni and saw something they, and many materials science researchers before them, had never understood before.
Instead of growing in a direction parallel to the magnetic field (akin to the expanding ink blob), the domains jetted out along precise, but seemingly arbitrary directions, which appeared to violate conventional notions of symmetry.
By incorporating fine details about the spin structure of the domain walls and a new interaction called the Dzyaloshinskii-Moriya Interaction, Sokalski and Kitcher, a Ph.D. student in materials science and engineering, were able to show with striking quantitative agreement that these directions were neither arbitrary nor a violation of the magnetic symmetry of the system.
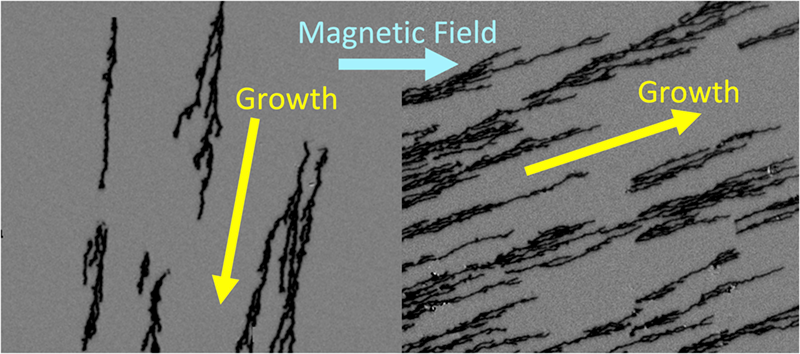
Source: College of Engineering
Images of expanding magnetic domains taken using the magneto-optical Kerr effect.
While this breakthrough will not directly give way to faster computers, it is a progression in understanding the fundamental physics of the technology that one day will.
Other team members involved in this research include Marc De Graef, a professor of materials science and engineering, and alumnus Maxwell Li. This research was published in Advanced Materials.