New modeling approach helps advance cryopreservation
Cryopreserving tissues and organs without damaging them requires a delicate dance through the principles of thermodynamics. CMU’s Biothermal Technology Laboratory has a new modeling approach for isochoric preservation to keep the work moving forward.
Prem Solanki and Yoed Rabin of Carnegie Mellon University’s Biothermal Technology Laboratory have developed a new way of modeling the solidification of fluids in confined volumes, with applications to organ and tissue preservation.
Cryogenics is the study, production, and use of cold temperatures. Cryopreservation is the study and practice of storing biomaterials at low temperatures. The field is almost 70 years old. Practical applications of cryopreservation include the preservation of stem cells, sperm cells, embryos, corneas, pancreatic islets, and other biomaterials. Cryopreservation is also used for biobanking plant seeds and tissues.
The potential of cryopreservation is enormous. Today, research on cryopreservation focuses on preserving whole organs for long-term storage and viability, the success of which can be a major medical advancement. Currently, organs like the heart, liver, and pancreas can survive for a few hours outside the body. Cryopreservation has the potential to extend this time limit to several days or even longer. “Ideally, we would like to preserve the organs for an indefinite period of time so that we have an organ bank from which we can solve a number of problems, including having a constant availability of organs and tissues on demand for transplantation,” says Solanki, a postdoctoral researcher in mechanical engineering.
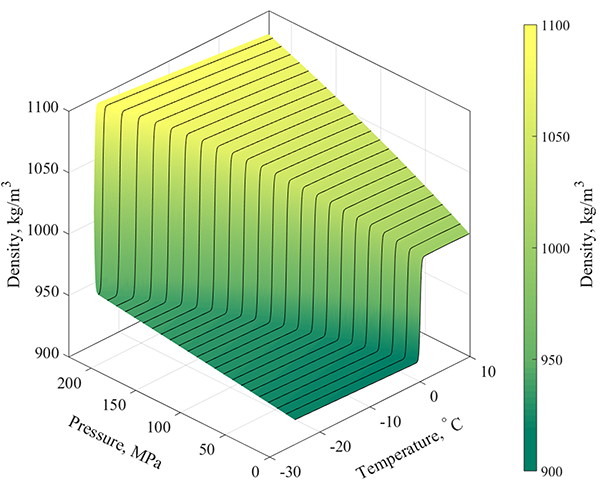
Source: Biothermal Technology Laboratory, Carnegie Mellon University
Density of water within the range of pressures and temperatures that the researchers investigated.
The field of cryopreservation moves at a glacial pace as the process comes with numerous challenges. When organic tissue freezes in uncontrolled conditions, it tends to die. The frozen region of the tissue stops receiving oxygen-rich blood, which quickly deteriorates tissue due to ischemic injury, an injury caused by lack of blood flow. At the microscopic level, ice crystal formation can burst apart cells, and at the macroscopic level, thermal stress can break blood vessels, both of which make the tissue unviable when thawed. To counter this, scientists replace the water in the tissues with a solution similar to antifreeze, known as “cryoprotective agents” (CPAs), allowing the tissue to be cooled below freezing without ice crystals forming. However, the ingredients in CPAs are often toxic to the organic tissue, requiring very particular concentrations of these ingredients in the solutions.
An alternative to the problem of ice formation is isochoric cryopreservation, freezing the tissue while maintaining a constant volume inside the chamber. “The idea of isochoric preservation is that you allow some ice formation such that the tissue specimen is preserved in the liquid part of the solution,” says Solanki. Ice formation occurs near the walls of the container, with the delicate tissue safely stored in the center. The solution expands in volume, but the rigid walls of the preservation chamber stop it. The only other option for the solution is to increase pressure, which reduces the temperature at which the solution freezes. This allows the tissue to be stored at below-freezing temperatures while still suspended in a liquid solution.
Isochoric preservation is what Solanki and Rabin, a professor of mechanical engineering, are studying. While isochoric preservation looks promising for the future of cryopreservation, it is currently extremely difficult to model mathematically. Ice behaves very differently from liquid water, and it is difficult to determine where ice will form in a system and how those “freezing fronts” interact with liquid water. The team’s latest research proposes a new method to model the isochoric cooling process that treats the frozen and non-frozen parts as one continuous pseudo-viscoelastic fluid.
This work focuses entirely on calculating these fluid behaviors in a system of pure water. This is very useful as a proof-of-concept. However, cryopreservation requires CPAs that behave radically different from water, especially when cooled below freezing temperatures. Some of these CPAs are unique to the field of cryopreservation. Because of this, their material properties have yet to be experimentally measured for use in computer models.
We are one of the very few engineering labs working in this domain.
Prem Solanki, Postdoctoral researcher and Ph.D. alumnus, Mechanical Engineering
“We are in the process of getting a lot of data from our collaborators,” explains Solanki. “We are one of the very few engineering labs working in this domain. So one of the big tasks we have in our lab is finding the material properties of these chemicals.” The team has already measured some of these material properties and recently published a paper supplying a database for the temperature-dependent densities and thermal expansion coefficients of some of these CPAs.
For now, cryopreservation has only been successful on very small tissue, such as embryos and stem cells. “When it comes to bigger specimens, there are still some challenges to face,” says Solanki. The largest pieces of tissue we have successfully preserved have been human blood vessels and there is research being conducted on preserving entire human ovaries. Solanki notes that we still need extensive research before we are able to preserve entire limbs and organs, but he is optimistic about the future of the field.