Discovery of naturally chiral surfaces for safer pharmaceuticals
Nisha Shukla and Andrew Gellman have developed a number of new, easier methods for making naturally chiral metal surfaces.
In the 1960s, the sedative thalidomide was widely popular as one of the only non-barbiturate, over-the-counter sleep-aids on the market. When doctors started noticing that it also helped alleviate morning sickness in pregnant women, many began recommending it to patients for this off-label use. What followed was a world-wide explosion in the numbers of children being born with phocomelia—shortened or absent limbs. Children whose mothers had been recommended thalidomide by their doctors.
Thalidomide was an otherwise harmless compound, so what caused it to have such deleterious side effects? The answer lies in the property of chirality.
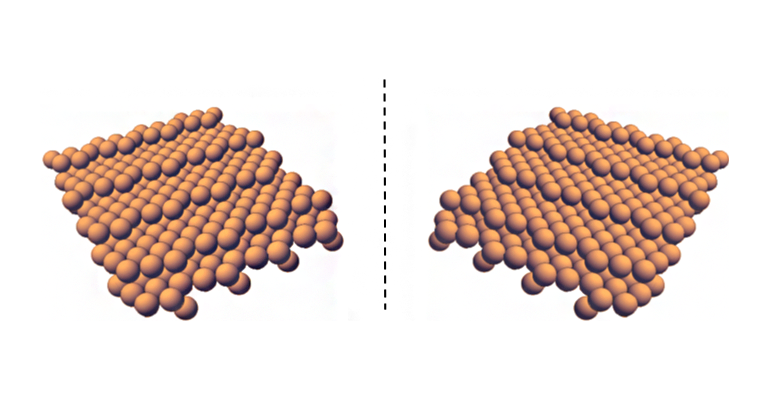
Source: Andrew Gellman
The two non-superimposable mirror images of a chiral metal surface at the atomic scale.
Much like a pair of hands, some molecules exist in two structures, known as enantiomers, that are distinguishable mirror-images of one another. These are called chiral molecules. These chiral molecules very frequently form the basis for many important pharmaceuticals. But when synthesizing these molecules for pharmaceuticals—pharmaceuticals such as thalidomide, ibuprofen, penicillin, and many more—it is very difficult to know which “hand” you’re going to get, and so manufacturers end up with batches of molecules that are a mix of both enantiomers. In contrast to these synthetic chiral molecules, the molecules that make up life on Earth are homochiral, meaning all sugars, DNA, amino acids, and proteins exist in only one of their two enantiomeric forms. Herein lies the problem: when chiral pharmaceuticals are manufactured without consideration of their “handedness,” one enantiomer may be therapeutic while the other is toxic.
So pharmaceuticals manufactures have a vested interest in being able to create batches of these chiral molecules and separate one hand from the other. For the most part, this is done by creating a surface out of chiral molecules of a single enantiomer. When you pass other chiral molecules over it, the homochiral surface molecules will grab onto those of their opposite enantiomer, trapping them on the surface, allowing only molecules of the desired enantiomer to pass successfully over. That way, what they are left with are molecules of only a single enantiomer, which can then be used in pharmaceuticals without running the risk of toxicity.
While many companies have managed to create their own artificial chiral surfaces for this purpose, Nisha Shukla and Andrew Gellman have developed a number of new, easier methods for making naturally chiral metal surfaces, as outlined in their perspective “Chiral metal surfaces for enantioselective processes,” published in Nature Materials.
“Until our original work, no one knew that metal surfaces could have structures that are intrinsically chiral,” says Gellman, a professor of chemical engineering. “But this discovery could offer new processes for doing enantioselective chiral chemistry and thereby enable new routes to enantiomerically pure chiral pharmaceuticals.”
Until our original work, no one knew that metal surfaces could have structures that are intrinsically chiral.
Andrew Gellman, Professor, Chemical Engineering
The majority of chiral surfaces in practical use, according to Gellman, are made out of achiral materials that are then treated or modified with enantiomerically pure chiral adsorbates, rendering them chiral and therefore usable in chiral separation. But this new research has shown for the first time that there are many routes to preparing inorganic materials, metals in particular, that are already intrinsically chiral, meaning they don’t have to be treated with these chiral adsorbates to be useful.
“These chiral inorganic materials are much more efficient than the traditionally used organic materials,” says Shukla, principal systems scientist in the Engineering Research Accelerator, “as they can maintain their chiral structure at higher temperatures and under conditions where chiral organic materials would decompose.”
But as with any commercial process, in order to be viable, it must be scalable. Through their research into these intrinsically chiral surfaces and analysis of recent developments in the field, Gellman and Shukla have shown that the path to scalable fabrication of these high-surface-area, enantiomerically pure surfaces is possible. In their research, they outline a number of potential methods for developing them—from growing chiral metal films, to imprinting surfaces with chiral patterns, to the development of chiral nanoparticles. They even present the possibility of impressing chiral surface orientations into textured substrates, though this will require further investigation.
“Each of these methods has the potential to bring the fully scalable manufacture of chiral metal surfaces to a number of industries, including pharmaceuticals, agrochemicals, and others,” says Gellman. “Which method is most efficient is ultimately dependent on the problems faced by that particular industry and the specific chiral chemistry a manufacturer is looking to control.”