Blast from the past
Developed in the early 90s, Michael Domach’s NMR spectroscopy reactor has been revitalized in research at the National Institutes of Health.
Vinyl records were it during the mid-1900s, until different ways to listen to music rendered them obsolete. But over the last decade or so, vinyl has experienced a resurgence in popularity. This is the way it works sometimes: what was once the ultimate experiences a fall from grace, only to be reborn to its previous glory. If you doubt, talk with Carnegie Mellon Chemical Engineering (ChemE) Professor Michael Domach.
Recently, the Laboratory of Cardiac Energetics—a research lab within the National Institutes of Health’s (NIH) National Heart, Lung, and Blood Institute—contacted Domach to adopt a nuclear magnetic resonance (NMR) technology he and a pair of former ChemE Ph.D. students developed in collaboration with Dr. Allan Koretsky and Dr. Beth Jones…in the early 90s.
“The lab has sort of decided they need to have more data for what they’re working on,” Domach says. “They came across this technology and thought it might be the recipe to get done what they’re trying to do.” What “they’re trying to do” is better understand how the molecules within cells are used to transfer and store energy. A clearer picture of this has the potential to influence all medicine, from preventative to prescriptive and everything in between.
NMR technology is most commonly encountered in magnetic resonance imaging, better known as MRI. Electromagnetic radiation (radio frequency energy) is transmitted into a subject and offers a picture of what’s going on inside that subject. In an MRI, the electromagnetic radiation produces a picture of what’s happening inside a patient’s body: for example, torn cartilage or tumors. Domach’s focus is on NMR spectroscopy—using a reactor to identify the abundance of different molecules within a cell and how they react in time.
Here’s how NMR works. When a subject—whether a chemical sample or a person—is hit with a burst of radio frequency energy, molecules within the subject that have “spin” (properties of magnetism) absorb the energy and get excited. Pretty quickly though, those molecules shoot the energy back out at a frequency that depends, in part, on the type of molecule. The energy being shot back out at different frequencies creates spectra (read: pictures) of the molecules present and their relative abundance at that instant. Once the molecules release that energy, they eventually relax back to the state they were in before the radiation burst. The process is repeated numerous times to gain a full readable spectrum by combining data from each individual radiation burst.
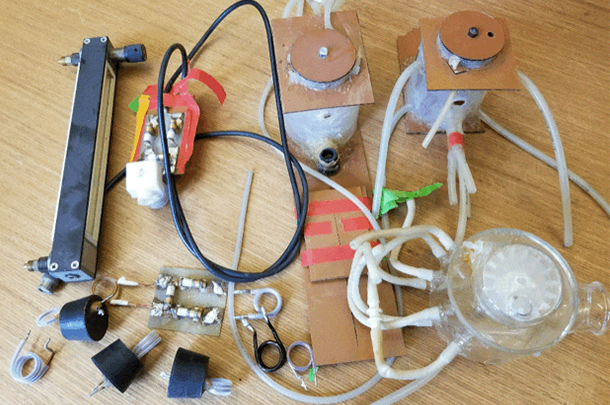
Source: Michael Domach
Disassembled parts of NMR spectroscopy reactor now with NIH
“The molecules have to sort of get back to what they were doing” before they can be hit with another burst of radiation, Domach says. “So that puts an automatic limitation on how fast you can get information out.”
This helps explain why the MRI process is so time-consuming. It’s also the reason NMR spectroscopy was traditionally ineffective in following molecular changes within a sample over time. During the time it takes for a sample to calm down, the molecules could be doing things that researchers can’t observe. Domach, his Ph.D. students, Anthony Meehan and Chris Castro, and Koretsky addressed this shortcoming by developing a spectroscopy reactor that uses chemostat system principles originally described by 1965 Nobel Prize winner Jacques Monod.
A chemostat is a controlled chemical composition system in which researchers manipulate the growth rate and abundance of cells by removing some cells and replacing them with new cells at a predetermined and constant rate. This creates what Domach calls a “steady-state little ecosystem.” In essence, every cell in a chemostat is growing at the same rate as chosen by the researchers. “We’re trying to replicate what a microbial physiologist would have done on the lab bench,” he says.
Domach combined chemostat system principles with fluid mechanics—how fluids move in response to forces on them—to create an NMR spectroscopy reactor that sidesteps the usual wait-time needed between bursts of radiation shot at a sample. To visualize Domach’s reactor, picture a coffee mug. Inside the mug are cells suspended in a liquid. The number and physiological state of the cells stay balanced because of the implemented chemostat system. An agitator keeps the composition well-mixed, while also guiding the liquid to flow through the handle of the mug, isolated and off to the side. When the liquid flows through the handle, it carries some of the cells with it. Those cells that end up in the handle are blasted with radio frequency energy, and each cell’s molecules absorb and return radiation, just like normal. But instead of waiting for those same molecules to calm down, the liquid flow carries them out of the handle and replaces them with cells that are ready to be blasted. Overall, a researcher can choose an initial growth rate for the cells, scan them for a baseline, and then perturb the system to watch the cells’ metabolic adaptations and responses.
For those folks, it’s all about the mitochondrial model and understanding how that sub-organelle works in heart and other cells.
Michael Domach, Professor, Chemical Engineering, Carnegie Mellon University
Domach shares that the NIH lab is looking to use his NMR reactor to analyze a microorganism called Paracoccus denitrificans. What makes this microbe so attractive to researchers is how similar it is to the mitochondria, the so-called powerhouse within cells that regulates respiration and energy production.
“What they can see then is what kind of molecules the cells are using to manage their energy metabolism,” he says. “For those folks, it’s all about the mitochondrial model and understanding how that sub-organelle works in heart and other cells.”
P. denitrificans can “do some pretty bizarre things and survive some pretty wild stuff,” he continues. Learning how, exactly, a microorganism so closely related to the mitochondria manages to survive events that should kill it gives researchers the opportunity to better understand things like ischemia, heart attacks, and oxygen loss.
With the chemostat system in Domach’s reactor balancing the cells’ environment, researchers can introduce the desired variables to see how the cells’ molecules respond. The real benefit, though, is the “real-time” view researchers get of how molecules work as time passes. Instead of waiting for molecules to recover, researchers have a constant fresh supply of cells with molecules ready to offer up information.
It’s taken a little time, but Domach’s NMR reactor is enjoying a resurgence more than two decades after its creation. Even as continuous innovation relegates the latest and greatest to the storage shed, nothing beats a classic at certain times and in certain instances. Teenagers setting a needle on a record for the first time aren’t the only ones learning that lesson. Researchers are too.