Shining a light on intracranial pressure
A non-invasive way to monitor patients with traumatic brain injuries.
Every year, millions of patients suffer from traumatic brain injuries (TBI) like concussions, brain contusions (bruises), hematomas (blood clots), and skull fractures—injuries often caused by car accidents, physical blows to the head, accidental falls, infections, and/or strokes. These patients must be closely monitored to make sure the pressure within their skull (otherwise known as intracranial pressure) doesn’t exceed a normal level. If this happens, their brain tissue could swell, which—if left untreated—could ultimately restrict the blood flow to their brain, causing major brain damage or even death.
To prevent a fatal outcome, doctors must monitor their patients’ intracranial pressure closely. But right now, the standard way to accurately monitor or measure intracranial pressure is by placing a probe in the brain—an invasive surgery that requires a hole to be drilled through the skull. For the past two years, biomedical engineering Assistant Professor Jana Kainerstorfer and her Ph.D. student Alexander Ruesch, in collaboration with Matthew Smith from the University of Pittsburgh, have been developing an alternative, non-invasive way for doctors to measure and monitor intracranial pressure using an approach based on near infrared spectroscopy.
Clinicians don’t have enough tools available to optimize treatment for their patients. I’m hoping we can develop a technology that can improve patient outcome.
Jana Kainerstorfer, Assistant Professor, Biomedical Engineering, Carnegie Mellon University
Ruesch and Kainerstorfer use near infrared light to measure and analyze the concentration of oxygenated and deoxygenated hemoglobin in the brain over a certain period of time. To garner data, they send near infrared light into the head via optical fibers. These light signals pass through the skin and skull before reaching the brain tissue, where they capture the behavior of both oxygenated and deoxygenated blood in the brain. A portion of the light is then reflected back and measured at the surface. With this measurement, blood volume changes can be monitored.
While conducting their initial studies, the duo discovered that they could use mathematical functions to translate their blood volume data into quantifiable estimates of intracranial pressure changes. If Ruesch and Kainerstorfer can use their system to gather blood volume data non-invasively, and if this data, in turn, can be translated into intracranial pressure measurements, doctors will no longer have to perform invasive surgeries to place pressure probes in the brain.
“That’s why this is so amazing,” says Ruesch. “If we can replace a very risky, invasive surgery and still get the information we need by shining light into a patient’s head, that would be much safer and much more cost-efficient. A non-invasive approach would be helpful for patients who are bed-ridden and need their brain to be constantly monitored. With our system, physicians won’t have to worry about jeopardizing the safety of their patients.”
The intracranial pressure measurements gathered from Ruesch and Kainerstorfer’s system will help doctors determine how well blood is flowing through the brain (also known as brain perfusion) and how well the brain can regulate this blood flow when intracranial pressure fluctuate.
With the help of a physiological mechanism called cerebral auto-regulation, the brain can regulate blood flow by expanding and contracting blood vessels when intracranial pressure increases. High pressure limits blood flow to the brain, preventing oxygen from reaching brain cells. However, with a functioning auto-regulation mechanism, the brain can increase the diameter of small arteries and make room for blood to flow. Thus, the brain can help facilitate blood flow during unexpected pressure changes.
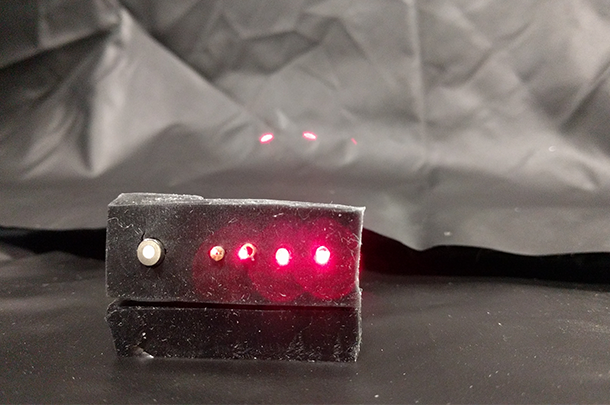
Source: Alexander Ruesch
A near-infrared spectroscopy probe showing the optical fiber tips of a detection fiber (on the left) and four light source positions (on the right). The source fibers emit two wavelengths of light, one at 690nm in red and one at 830nm in the invisible, near-infrared range.
“You always want this mechanism to be active,” says Ruesch, “but in diseases like stroke, hydrocephalus, and traumatic brain injury, this mechanism can be impaired. If your brain can’t regulate blood flow when intracranial pressure increases, your brain could stop working properly, which can be extremely fatal.”
With Ruesch and Kainerstorfer’s system, doctors can determine if the cerebral auto-regulation mechanism is intact by first non-invasively monitoring and measuring a patient’s intracranial pressure and then comparing that data to their blood pressure at certain points in time. When doctors notice an increase in intracranial pressure, they want to see a subsequent drop in blood pressure because that means their patient’s blood vessels are properly expanding to make more room for blood to move and transport oxygen and nutrients. In other words, that means their patient’s auto-regulation mechanism is working.
Fortunately, with Ruesch and Kainerstorfer’s system and non-invasive data, doctors will be able to recognize when their patients’ auto-regulation mechanisms aren’t working properly, which will help them maintain the proper balance between their patients’ blood pressure and intracranial pressure by, for example, draining cerebrospinal fluid.
To continue work on their project, Kainerstorfer and Smith have received funding from the National Institutes of Health (NIH) and the American Heart Association (AHA). Within the next month, they hope to translate their methods to the Children’s Hospital, where they will use their system to monitor pediatric patients suffering from traumatic brain injuries.
If we can replace a risky, invasive surgery and still get the information we need by shining light into a patient’s head, that would be much safer and much more cost-efficient.
Alexander Ruesch, Ph.D. student, Biomedical Engineering, Carnegie Mellon University
“The grant from the American Heart Association is focused on trying to understand if intracranial pressure is changing, how the brain regulates blood flow, and how we could use non-invasive methods to measure how well the brain regulates that blood flow,” says Kainerstorfer. “Whereas the NIH grant is more focused on translating our non-invasive measurements of blood volume in the brain to intracranial pressure as well as understanding the impact of pressure on neurons.”
Overall, Ruesch and Kainerstorfer are searching for a way to better serve patients with traumatic brain injuries. “What we’re ultimately trying to do is provide insight into what’s happening in the brain for patients suffering from diseases like stroke and traumatic brain injury,” says Kainerstorfer, “because right now, clinicians don’t have enough tools available to optimize treatment for their patients. I’m hoping we can develop a technology that can help manage patients and improve patient outcome.”