Big impact, tiny element: hydrogen power, amplified
Ajay Pisat hopes to unlock hydrogen’s full potential as a primary, mainstream energy storage medium by maximizing the efficiency of hydrogen production through photocatalysis.
Using hydrogen for energy production is nothing new. But with his research, Materials Science and Engineering (MSE) Ph.D. candidate Ajay Pisat hopes to unlock its full potential as a primary, mainstream energy storage medium by maximizing the efficiency of hydrogen production through photocatalysis.
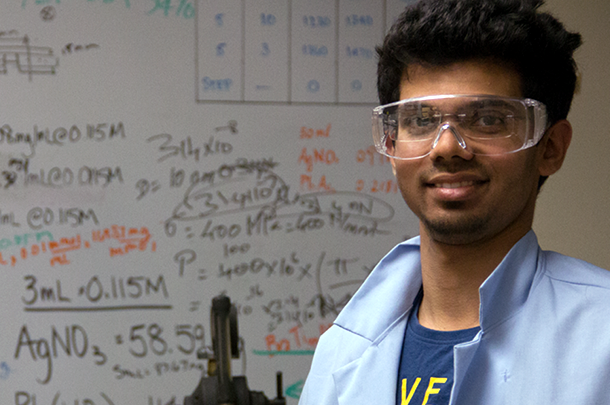
Source: Carnegie Mellon University College of Engineering
Ajay Pisat hopes to unlock hydrogen’s full potential as a primary, mainstream energy storage medium by maximizing the efficiency of hydrogen production through photocatalysis.
While there is much research being done with photocatalysis, Pisat’s work at the nano-level is an important first step leading into larger scale research, because it focuses at the lowest possible level, the structural level. He spends his time in the lab engineering the surface morphology of oxide compounds, optimizing their surface areas for hydrogen evolution which, in turn, increases the efficiency of hydrogen production through photocatalysis.
If Pisat is able to scientifically engineer the surface structure of the oxide compound his lab uses—strontium titanate, which has a similar structure to many other oxide compounds—for maximum efficiency, then he can engineer similar compounds which absorb sunlight better. Researchers could then use these compounds at a larger scale, on entire photocatalytic systems.
“We feel that it’s the structure of the material which is vital to the process,” Pisat says. “But the chemistry of the material is vital to the actual functionality.”
The science
Photocatalysis, in general, is using light in conjunction with catalyst materials to enable or quicken chemical reactions. The particular reaction involving the splitting of water into hydrogen and oxygen is called photocatalytic water splitting, or photocatalytic hydrogen production. The hydrogen produced can then be used to power on-site fuel cells or generators that are independent of infrastructure-based power grids—in other words, a consistent power source in places where those power grids don’t reach.
Two additional benefits of continued hydrogen power research are the cleanliness and renewability of the resulting hydrogen power. Unlike the carbon emissions and greenhouse gases that result from fossil fuels, hydrogen power does not yield harmful byproducts.
Additionally, the sunlight and water required for photocatalytic hydrogen production are nearly infinite. Along with sunlight and water, photocatalysis requires a catalyst. A catalyst is a material that increases the rate of a chemical reaction. In the photocatalytic process, a catalyst (most commonly an oxide compound) is submerged in water. When the water is bombarded with sunlight, the catalyst causes a chemical reaction at the points where the catalyst compound contacts the water. It is this reaction that splits the water molecules apart.
While this may seem like a fairly straightforward process, researchers have encountered a few roadblocks that keep photocatalysis from producing its maximum potential of hydrogen. One is the light absorption of the oxide compound catalysts. To date, scientists have struggled to find an appropriate compound that is able to absorb the visible range of the solar spectrum, which holds the energy needed for photocatalysis. Most compounds are very good at absorbing UV rays, but those rays account for only 5% of the entire light spectrum, and some compounds absorb infrared radiation, which does not have sufficient energy for photocatalysis.
Another challenge is the amount of hydrogen produced by any one catalyst. Hydrogen production is directly related to the surface area of the catalyst.
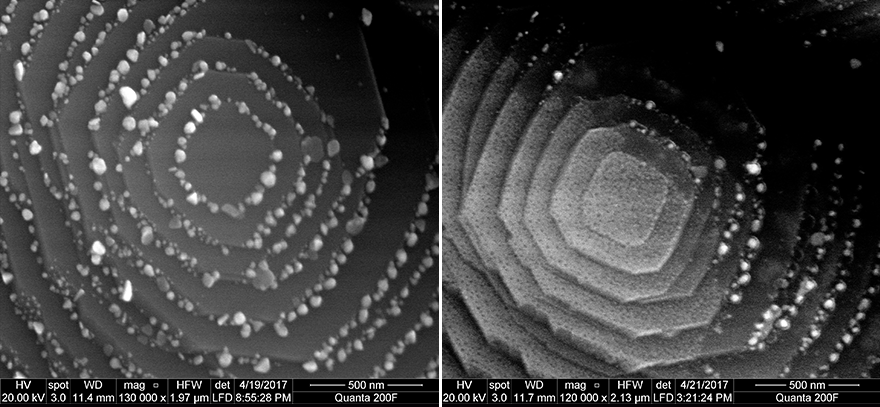
Source: Ajay Pisat
These top-down micrographs of Pisat’s samples show that different reactions occur on different parts of the surface. “To put it into perspective,” says Pisat, “a human hair is about 100 microns in diameter. So, this is 1/50th the size of human hair.” If you look closely, the “surface” looks like it is made of circular plates—these are called “terraces.” The silver particles have deposited around the circumferences (the edges) of the terraces in the first image, and the lead oxide is the porous, spongelike substance that has deposited on the top, flat parts of the terraces in the second image.
The way Pisat explains it: imagine your cellphone submerged in a tub of water. Everywhere the water hits the outside of your phone is where chemical reactions (e.g. hydrogen production) will occur. Now image cutting your cellphone into two pieces.
“Now we have exposed two more surfaces,” Pisat says. “And if we keep doing this, we are just going to expose more and more surface for the same amount of mass.” Since water is contacting a greater amount of the surface of the phone, more hydrogen will be produced.
Now try to imagine something smaller than a phone. Maybe, for instance, a cup full of pellets so small that they look like powder. Imagine picking up just one of those pellets. It’s almost like picking up a grain of sand, only smaller. This is where Pisat does his work: the nano-level.
Photocatalytic reactions actually consist of two individual reactions: hydrogen evolution and its counter reaction. Different surface structures tend to favor one reaction over the other, reducing the efficiency of the overall reaction. Pisat’s nano-level work is about balancing the areas of those individual reactions using inexpensive thermal treatments so the overall reaction can proceed as efficiently as possible.
The inspiration
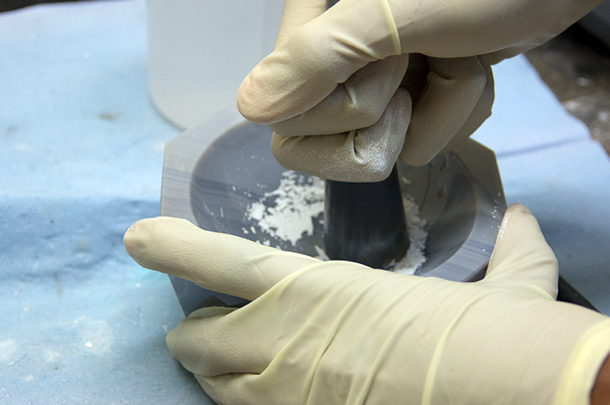
Source: Carnegie Mellon University College of Engineering
Ajay Pisat uses a mortar and pestle to crush a chemical catalyst, increasing the surface area of the catalyst to maximize the amount of hydrogen produced in the subsequent photocatalytic reaction.
Though his research is singular and amazingly focused, Pisat understands his work as just one small piece of a much bigger process. That bigger process might aptly be described as a six-lane superhighway, each lane barreling towards the same finish line. It just so happens that Pisat found his lane as an undergraduate in India.
As a native of Mumbai, he grew up in a world that influenced his decision to pursue the research he has. “In my own lifetime,” he says, “I got to see that the climate is not the same. I have seen the quality of air degrade throughout my own childhood.” Every five years, he shares, he could see and feel the quality of the air lowering around him.
So when he entered college, he was very pointed about the major he chose: materials engineering. Through his studies, he became involved with research on clean, renewable hydrogen power through photocatalysis and the material engineering of oxide compounds.
In pursuing his Ph.D. at Carnegie Mellon, he chose a program where he could study with not one, but three professors conducting nano-level research on oxide compounds used in photocatalysis: Gregory Rohrer, Paul Salvador, and Mohammad Islam. Currently, he is being co-advised by professors Rohrer and Salvador.
Taking a broad view of his research and that of others, Pisat appreciates the work that still needs to be done.
In my own lifetime, I got to see that the climate is not the same. I have seen the quality of air degrade throughout my own childhood.
Ajay Pisat, MSE Ph.D. candidate, Carnegie Mellon University
“It might not be used straightaway because everything else is just so cheap,” he says, when asked about the practical future of hydrogen power. “Coal is just so cheap. So competing with those kinds of technologies is going to take some time.”
While, as he says, it will take some time, Pisat has made great strides. “I’m definitely making a lot of progress,” he says, in terms of the results he is seeing in the lab. “Once this technique [surface morphology optimization] is perfected,” he adds, “people are going to try to use it to engineer entire photocatalytic systems to make hydrogen production completely driven by solar power. Maybe then, after five or ten years, we can see them competing with gas.”